Reductionist Magic 7.2: Biochemistry of ectoplasm
Chemistry and biomechanics of ectoplasm and unsnapping reactions
Continuing from my previous article in the series, this time I will discuss the chemistry and biomechanics of ectoplasmic atoms. Long story short - what happens if you eat a summoned horse?
As usual, if you are confused about any terms, make sure to check out the glossary (though terms in this article will be mostly chemical, and so wikipedia will serve you better), and if you are looking for other posts, check out the blog map. I would also like to thank Augsphere and Verglasz for various help on this article.
1. Ectoplasmic chemistry
Let’s start from the ground up, and consider how ectoplasmic atoms interact with chemistry. I have already described a couple reactions in the previous post, but this time, we will be dealing much more closely with these concepts. This means we will have to define our notation more strictly. Looking at an example of a chemical reaction involving ectoplasmic matter would be instrumental here.
The entire life cycle of summoned matter is outlined here. First, some coal appears (line 1). It is burned with oxygen, releasing heat and carbon dioxide (line 2). Then carbon disappears (line 3) leaving two unbound oxygens. Finally, free oxygens recombine into an oxygen molecule, releasing yet more heat (line 4).
The reaction in line 1 is not chemical as it does not involve any other compounds, and has already been discussed in the previous post. The only thing worth mentioning here is a list of typical compounds summoned by the various spells. Reactions in line 3 (unsnapping) and line 4 (recombination) are the core of what makes summoning chemistry unusual.
Reaction in line 2 is completely normal chemistry and so is not very interesting to us from a mechanical perspective. It is nonetheless essential to discuss what typical chemistry happens to typical summoned compounds, as this determines the inputs to reactions 3 and 4. After all, there are infinitely many potential molecules you can make where at least one atom is ectoplasmic, and so the space of potential unsnapping and recombination reactions is all but infinite; but we can ignore most of that space because it would not happen in practice.
1.1. Typical summoned compounds
Despite the great potential inherent in the idea of summoning matter, in practice most spells only utilize a fraction of the whole possibility space. This is due to two factors: the origin of TTRPGs in general and DnD specifically, and the typical rules design process utilized by the writers.
DnD is named after dungeons. It was, in fact, one of the first (if not the first) dungeon crawlers on the market. As such, rules for it are mostly concerned with exploration of dungeons, combat, and various adventure utilities. Therefore, summoning spells mostly summon various weaponry, meatshields, and camping supplies, as opposed to something far more exotic.
This problem is further compounded by the fact that designers rely on their intuitions when designing spells. This isn’t unusual, and is inherent to any kind of creative process. But the vast majority of designers are not going to be chemists or physicists. Their intuitions will be informed by what they see around them in their lives, or what they see in other media. This means that it will be harder for them to imagine the sheer space of possibilities inherent within chemistry and physics, and so their designs will tend to underutilize the options logically available to them.
Based on the spells available, I would classify the summoned compounds into five groups:
Water. Spells like Drench, Icicle Dagger, Aqueous Orb, Sleet Storm, Geyser or Tsunami create (mostly) pure water.
“Acids”. Spells like Acid Splash, Acid Arrow, Acid Pit or Acidic Spray all create something highly corrosive. Whether it’s a real acid or not remains to be determined - for example, it tends to work far too quickly in comparison to any acids available in reality.
Metals and Silicates (aka rocks). On the metal side these are spells like Major Creation and Pellet Blast, and on the silicate side it’s Stone Call or Secure Shelter, as well as Wall of Lava.
Oxygen and oxidizers. Air Bubble is a notable example of a permanent air conjuration spell. Additionally, this category would include fire spells (e.g. Fiery Shuriken) that follow the mechanism described in the previous article.
Organics. This comes in various forms - Summon Monster type spells summoning organic minions, as well as spells like Web, Conjure Carriage or Minor Creation summoning various organic matter. All of these spells summon something primarily organic, meaning long carbon-based molecules, with hydrogen, oxygen and nitrogen as the other primary elements. See composition of the human body as an example of the relative elemental composition you can expect.
1.2. The acid problem
As I mentioned above, summoned acids tend to be too strong compared to normal acids. For example, Caustic Eruption can deal up to 20d6 acid damage. If we convert it into a more reasonable metric, such as the time needed to dissolve an inch of metal, we can get the following graph:
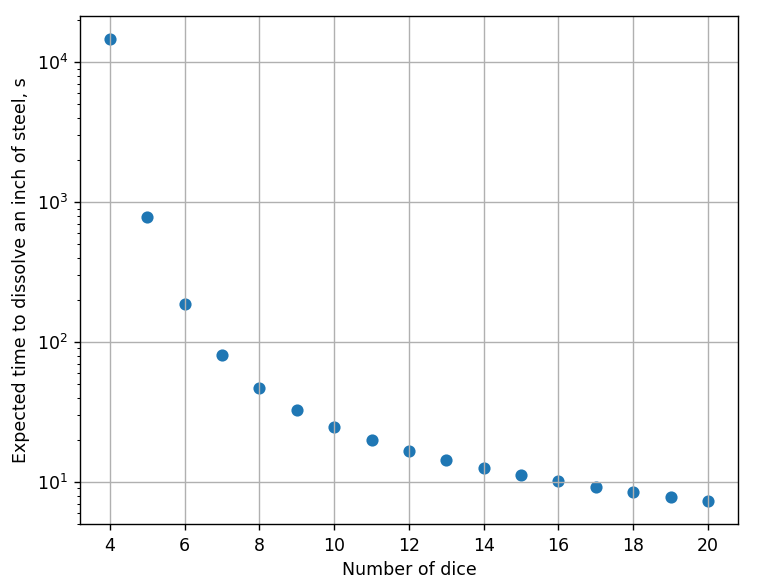
As we can see, we start out at a fairly reasonable amount of time (~3 hours), but 5 dice of damage already gives only ~20 minutes, 6 is ~3 minutes, and 7 is only about a minute and a half. By the time we are dealing with 20 dice of damage, we are looking at sub-10 second dissolving time.
This is a problem. Normal acids deal damage by oxidation: it’s the same sort of reaction as with oxygen, except with an acid. This reaction releases heat. The faster this reaction goes, the more heat it releases; a reaction that would go fast enough to dissolve an inch of steel in ten seconds would also be violent enough to set everything around it on fire. This is why very strong acids are a classic kind of rocket propellant. Fast-burning metals are also used for that purpose. That, in turn, would mean that large amounts of acid damage should actually be fire damage; and it would not look like your flesh melting off, it would look like you suddenly bursting into unquenchable flames.
I don’t think there is any easy way to square this circle chemically. You can either have chemical acids that look at least a bit similar to acids in popular media; or you can have acids that dissolve an inch of steel in seconds, but not both.
In order to have something that looks like a movie acid and acts fast, we need magical effects. I see several options here:
Self-cooling acid. The reason acids burst into flames is that the heat generated by the reaction is sufficient to produce plasma, and thus visible flame. If you apply magical cooling as the acid reaction happens, you can avoid this problem, thus (theoretically) letting you have your cake and eat it too. I don’t have sufficient chemical knowledge to say wherever this is actually feasible, and obviously nobody does experiments on this, so use at your own risk. This solution is somewhat self-defeating: generally, the warmer compounds are, the faster a chemical reaction goes, increasing by a factor of 2-3 for every 10 degree increase in temperature.
Acid + a matter grinder. In principle, to achieve the same visual effect, you don’t need to dissolve 100% of the material. You only need to dissolve enough to render the object effectively unusable. An easy way to do that is to add some microscopic force walls to the acid’s acting mechanism, that would grind any material in contact with the acid into crumbs, thus speeding up the corrosion process greatly. Crumbs themselves would not be completely corroded, but it won’t matter as much. Acid itself would act as a carrier for the force wall effect, and apply corrosion on a more fine-grained scale. Discussion of force walls would have to wait until their own post.
Transfigurative acid. At this point, we have abandoned the entire idea of chemistry: acid is simply some kind of magical liquid. In this model, acid permanently transmutes flesh into some other liquid on contact; this, in turn, leads to visible corrosion. Discussion of transmutation would have to wait until it’s own post, but for now, I can say that you would need to consider chemical reactions between the result of transfiguration and surrounding materials, as well as potential volumetric changes (e.g. when 1 liter of flesh is turned into 100 millilitres of liquid X), and their impact on environmental pressure.
Disintegrative acid. Instead of transfiguration, acid disintegrates material it comes into contact with. Same as transfigurative acid, you would need to consider how quickly this reaction happens; furthermore, you would need to figure out how you can store such an acid, since acid flasks are an item in the core rulebook. I can see three logical options. First, it could be a two-component mixture (two things that turn into an acid when mixed, but are safe separately). Second, there could be a second magical material that is immune to the disintegrative effects of acid (and would thus be used in storage containers and in antacid armor). Finally, you could say that this type of acid is only produced by high-level spells and cannot be stored; acid flasks use a different type of acid. Same as transfiguration, pressure effects need to be considered.
For now, I’ll consider summoned acid to be acting chemically. More exotic material types would deserve their own post.
1.3. Typical chemical reactions
Now that we have a list of the basic types of summoned matter, let’s consider what compounds we might get as a result of chemical reactions.
Water is mostly non-reactive chemically speaking. Overall, I only see three reactions that are simple enough to come up in play.
Electrolysis is a classic reaction where water is split into oxygen and hydrogen under electric current.
Alkaline metals react with water to produce bases and heat.
Alkaline earth metals react with water to produce oxides and heat.
This is also a natural place to introduce hydrogen’s reaction with oxygen to form water, as it’s a common byproduct of various reactions.
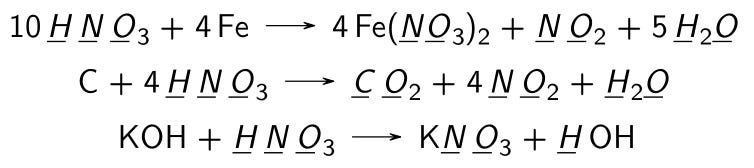
Acids. As I have said in the previous section, I will consider acids to be chemical for the rest of this article. This means these reactions would not be applicable for any spell that you determine uses a more magical form of “acid”.
Metals react with acids to form salts, water, and (potentially) oxide corresponding to the acid anion.
Carbon reacts with acids to form carbon dioxide, oxide of the acid element, and water. Naturally, this reaction doesn’t happen when the acid doesn’t have oxygen in it (e.g. hydrochloric acid being just H Cl).
Bases react with acids to form salt, and partly-ectoplasmic water. Note that unlike the note I have made in the previous article regarding hydrogen exchange, this water will not be fully ectoplasmic - there is no free ectoplasmic oxygen being generated. Most common place for an acid to encounter a base is probably lye - a key ingredient in soap making.
Organic reactions with acids are complex, depend to a large degree on the type of acid being involved, and so I won’t draw them here. In general, you can expect organic molecules to break up rapidly into smaller chunks and form compounds with the acid anion. All of that will release heat.

Metals
Oxygen can burn metals, producing metal oxides. This process happens on the surface of all metals; in some metals (e.g. aluminum) a film of oxide has the same volume as the metal, and so remains on the surface, protecting the rest of the object. In others (e.g. iron), oxide film flakes off, and all of the metal will corrode over time.
Acids oxidize metals into salts, in a reaction we have already overviewed in a previous section. This reaction often times releases hydrogen gas, which in this case will be permanent, and in the case of ectoplasmic acids, ectoplasmic.
Silicates (aka rocks) are mostly chemically immune, because they are, as a rule, already oxidized. You can’t really oxidize them further without a lot of effort, and so they generally don’t really do much.
In addition to chemical reactions, both metals and silicates can be melted, and then mixed with other metals and silicates. This would produce a solid chunk of material, indistinguishable from normal; but, invisibly, ectoplasmic atoms would be mixed in. What happens afterwards will depend on how well the mixture was mixed in a liquid state; you can get anything from fairly separate phases with some diffusion at the contact surface, to a fully intermixed normal-ectoplasmic alloy.
Oxidizers
Metals can be oxidized by oxygen, as previously mentioned.
Hydrogen can react together with oxygen to form water, in a reaction that has been noted a couple times already.
Carbon is a classic example of something being burned, producing carbon dioxide or monoxide.
Organics being burned can result in hundreds of different compounds. Generally though, you are going to be looking at a lot of carbon dioxide, water vapor, oxides of various atoms making up whatever you burned, as well as soot - incompletely burned pieces of carbon or organic molecules.
Organic matter
I have already described reactions of organic molecules with oxygen and acids, so I will not copy them here. The only key difference is that a different set of atoms is ectoplasmic - ones coming from organic molecules as opposed to oxygen and acid.
Heat alone can be used to break up organic matter; this is how charcoal is made. This happens through a chemical reaction known as cracking (no relation to the mining method, best as I know). Outcomes of cracking depend heavily on what you are using as the source and how far you are going with it, but generally, you can expect to find carbon, hydrogen, simple hydrocarbons like methane, water vapor, carbon dioxide (from the oxygen present in organic molecules), calcium and phosphorous (bones and part of organic molecules), and other simple compounds.
1.4. Unsnapping reactions
Unsnapping reaction’s products depend solely on the structure of the molecule which contains ectoplasmic atoms. To determine them, you simply have to look at the molecular structure of the partially-ectoplasmic compound and remove all the ectoplasm. For example, for sulfuric acid with ectoplasmic sulfur, this reaction has the following form:
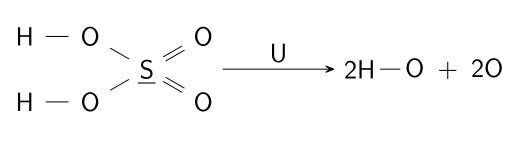
Here I will note some typical unsnapping reactions, based on the resulting compounds discussed in the previous section. Naturally, if the entire molecule is ectoplasmic it vanishes without a trace, and there is no point in discussing it.
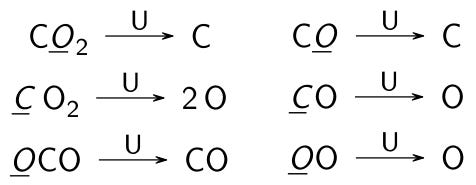
Carbon dioxide ...
...with real carbon leaves behind pure carbon, which would be either oxidized in air to carbon dioxide, or would fall to the ground as very fine graphite/coal dust, depending on how hot it is already.
...with real oxygen would leave behind two atomic oxygens. They would either oxidize one another to O2, or will rapidly oxidize the first thing they find. In either case, lots of heat will be released.
...with only one ectoplasmic oxygen would leave behind carbon monoxide.
Carbon monoxide forms either oxygen or carbon, with consequences similar to those of carbon dioxide.
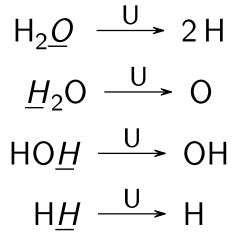
Water …
...with ectoplasmic oxygen produces two hydrogen atoms that would immediately try to find something to form a molecule with. Given their relative closeness, it’s likely they would form normal hydrogen.
...with ectoplasmic hydrogens would form an oxygen atom, same as one of the reactions in the previous section.
...with only one ectoplasmic hydrogen would form a hydroxyl radical. This is a relatively stable gas, though it reacts with most things to produce something more stable, and decays into water and oxygen when condensed.
Metal compounds
Salts (easiest formation pathway being acids reacting with metals) would generally break up into pure metal, or acid radicals (in this case, nitrate radical), which would (typically) swiftly decompose into oxides and oxygen.
Oxides have two breakup pathways: into pure metal (or a lower-rang oxide, if some of the oxygen is real), or into oxygen radicals
A brief mention should be given to organic molecules that have some ectoplasmic atoms as part of their structure. Generally, this leads to two consequences.
If the vanishing atom was part of the core structure of the molecule, it would get broken in half, and thus would likely stop working as intended. If it was part of a side branch, the molecule would get “merely” deformed, which may still stop it from working as intended.
Molecule is radicalized: regardless of wherever the vanishing atom was key to the structure, it is likely that the molecule now is in an unusual high-energy state, similar to how atomic oxygen or hydrogen is unusual. It would try to join some other molecule in order to reduce this potential energy, thus damaging other molecules nearby.
1.5. Recombination reactions
Recombination reactions depend on the compounds left after unsnapping, and what is available in the immediate environment. Key value determining wherever a given reaction would happen is enthalpy - to simplify, if the difference between enthalpy of the result of the reaction and the sum of the enthalpies for reactants is below zero, reaction is happening, and if it’s above zero it does not. This is not quite true, but will suffice for our purposes. It is, in truth, mostly irrelevant because our recombination reactions are generally energetic enough they will react with anything and everything that is nearby. If you need a big table of enthalpies, I have found Carl Yaws’s Chemical Properties Handbook to be useful.
To make it easier to look up the corresponding reaction, I have first grouped things based on the major recombining compound, and then based on the molecules that may be present nearby. To figure out the reaction in a real environment you would then need to figure out what molecules can be present nearby (e.g. mostly H2O in water, and 20/80 mix of O2 and N2 in air), and then look up the reactions for those molecules.
O, atomic oxygen. Formation enthalpy 249 kJ/mol. So strongly reactive that it can force a reaction with basically anything. Mostly produced from CO2 and H2O with ectoplasmic atoms.
Together with itself forms oxygen or ozone; together with regular oxygen forms ozone.
Together with nitrogen forms nitrogen oxides; Enthalpy basically doesn’t matter here, as atomic oxygen can force a reaction with all of them.
Together with water forms hydroxyl radicals, a somewhat less reactive oxidant.
Oxidizes organics, breaking apart their molecules into smaller ones, down to carbon dioxide.
Oxidizes metals into oxides.
Oxidizes carbon into CO and then into CO2.
If produced from CO2, it is likely to recombine and form O2 and a lot of heat due to proximity of two oxygen atoms to one another.
H, atomic hydrogen; Formation enthalpy 218 kJ/mol. Produced mostly from water with ectoplasmic oxygen in pairs.
Hydrogens are the opposite of oxygen: where oxygen is trying to steal some electrons from another molecule, hydrogen is desperately trying to donate it’s one electron. The end result is the same: desperate desire to recombine with other atoms or molecules to form a molecule.
Due to initial proximity of two hydrogens in the water molecule to one another, they will likely recombine together to form H2. This is not a certainty, though.
If hydrogens are left to wander around alone near organic molecules, they are likely to attach themselves to the first organic molecule they find, turning double bonds into single ones and lone O groups into OH. This would probably make the molecule non functional for its original purpose; overall, a similar sort of situation to molecular oxygen.
Hydrogens in air will recombine with oxygen to form water (-240 kJ/mol), or with nitrogen to form ammonia (-46 kJ/mol).
HO, hydroxyl radical. Formation enthalpy 37 kJ/mol.
Because hydroxyl radicals are apparently formed easily under radiation, a decent amount is known about their reactivity. Some sample reactions can also be found on wikipedia.
For organics, it does the same thing as oxygen and hydrogen: damages the molecules in significant ways.
Pure metals; Produced mostly from acid salts and oxides.
React with oxygen to form oxides. Reaction speed depends on the size of the surface area, concentration of oxygen and the temperature of the metal.
If recovered from a semi-ectoplasmic salt, metal atoms would not be connected to one another and would form an atomic suspension until they fall to the ground. Their surface area would thus be maximized.
If melted down together with normal metal and allowed to solidify, it produces voids filled with vacuum inside of the material. If well-mixed, voids will be broken up and small; this will manifest as a huge reduction in fracture strength, making it much easier for fractures to manifest and spread, similar to what happens after severe cases of radiation damage. If not so well mixed, voids will be concentrated in some parts of the material, and will link together; the larger the void, the bigger the problem this could be. If such a void connects to the surface of the material, it will fill with air; otherwise, it will grow as metal gradually vanishes during the unsnapping process, until metal buckles inwards implosively with great force as it can’t hold the pressure difference between the environment and the the vacuum pocket inside. In either case, the effect will be equal to a large chunk being taken out of the object.
If ectoplasmic metal is really well intermixed with normal, I think that the resulting material will be some sort of metal sponge or aerogel, due to the millions of tiny pores. If formed in air, likely result will be that it would collapse, as the air won’t have enough time to percolate into the depths of the sponge structure.
Carbon is produced mostly from ectoplasmic CO2 where oxygens vanish. It reacts with oxygen to produce CO2; needs to be preheated to about 150C for this reaction to start. Thus, if it is formed as a result of ectoplasmic CO2 dissolving, which in turn was formed from a combustion reaction, it will instantly combust in air.
1.6. Consequences of recombined compounds
Oxidative stress. Atomic oxygen produced from CO2 or H2O is a very active oxidant that will try to bond to anything it touches. If this happens inside your body, this will lead to oxidative stress - a general term for various compounds in your body being oxidized when they shouldn’t be, causing various damage. Your body has methods for repairing this damage, so the degree of severity of this problem would depend on whether you would overwhelm the recovery mechanisms or not. I think that oxidative stress would also be a general consequence of various organic molecules breaking apart due to some atoms in the middle vanishing - almost certainly parts of those molecules will try to bond to something.
Two-phase burns. Atomic oxygen from CO2, or hot carbon from CO2 in the air would initiate an unexpected fire when unsnapping happens. Let’s assume an ectoplasmic candle is burned, producing CO2. That hot gas would fly off to the ceiling and then diffuse throughout the room. When unsnapping happens, atomic oxygen thus formed would oxidize whatever is nearby; if it is concentrated enough, it may start new fires. If the concentration is not sufficient, likely nothing visible would happen. If the unsnapping delay is relatively short, you might see a fire starting just above the candle on the ceiling.
Hydrogen stress. Not something that happens in regular chemistry much, but in our case, an ectoplasmic oxygen vanishing from the middle of a water molecule would lead to production of two atomic hydrogens. If those hydrogens won’t recombine together into H2, they will cause an effect similar to oxidative stress, by attaching themselves to random molecules. On the other hand, H2 itself may actually be helpful against normal oxidative stress, though evidence is somewhat sketchy.
Pungent smell. Atomic oxygen in air immediately produces ozone, as well as nitrogen oxides; all of those smell extremely sharply, and so would anything that produces atomic oxygen. Mages who work with such conjuration spells would likely recognize the smell very easily.
2. Glycolysis and the Krebs cycle
There are two chemical pathways which I decided to consider separately instead of as part of the previous section. The first such pathway is glycolysis, followed by the Krebs cycle. This is what converts food molecules (e.g. glucose) into usable energy inside of your cells. I won’t discuss how various molecules may be converted into glucose, and will just take it as given: otherwise this article would turn into a whole book.
I am assuming that glucose comes from some summoned food that was ingested, so it is entirely ectoplasmic. I am further assuming that whatever processes precede glycolysis have already happened with no side effects.
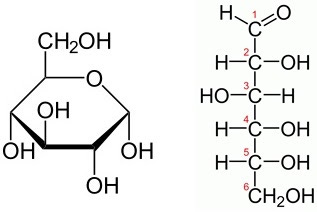
2.1. Key transformations
Before we get into a discussion of the glycolysis pathway, let's get some key chemical transformations out of the way, which will be used all over the place in this section. The first one is transformation of ATP into ADP and back:
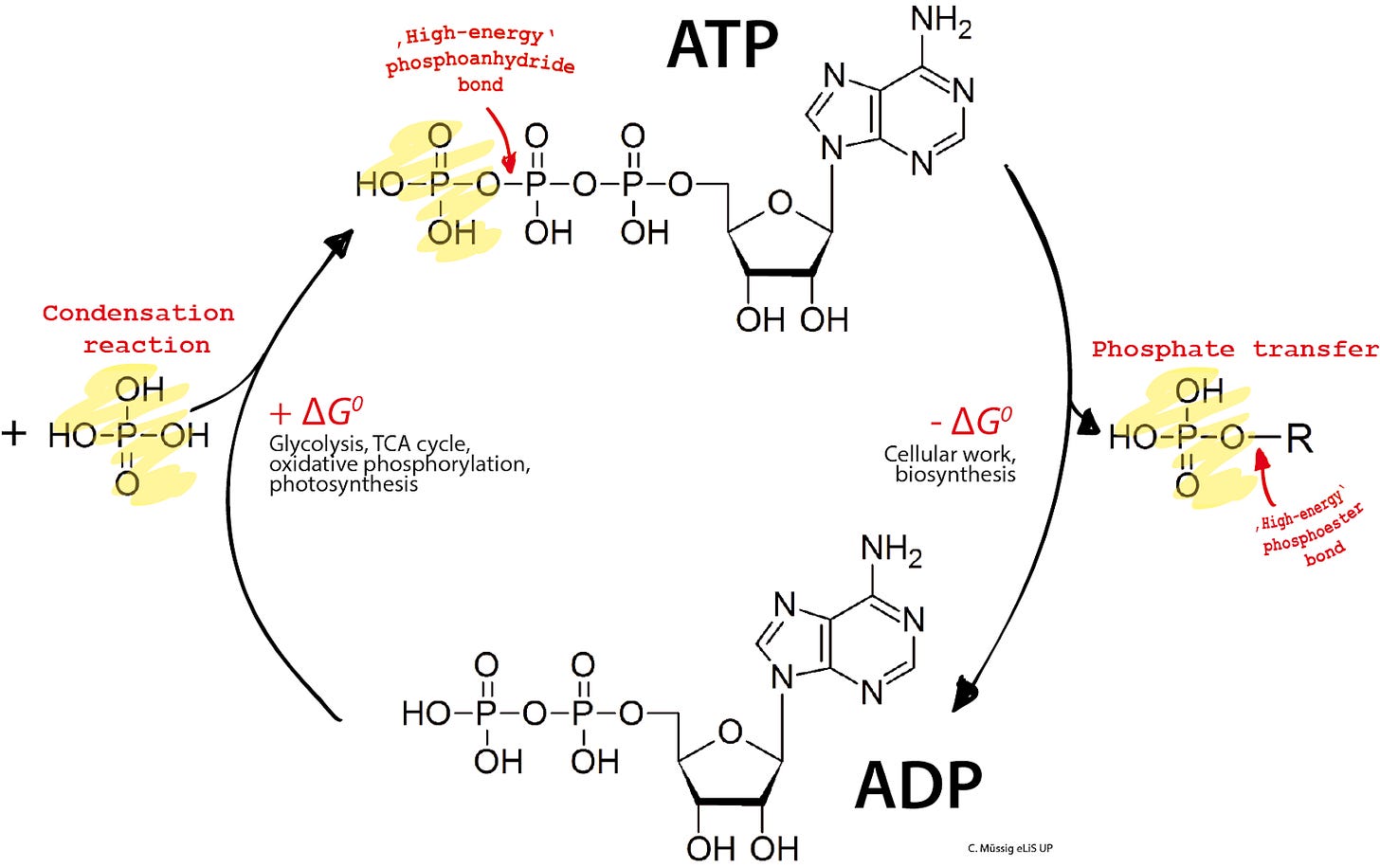
As you can see, all that is happening here is that a phosphate group is added or removed from the base molecule. This phosphate group is called Inorganic Phosphate, and is present in the cytosol of cells, just freely floating in some concentration. What is not shown is a single water molecule, which is released during ADP -> ATP and consumed during ATP -> ADP. Alternatively, ATP->ADP reaction can borrow an OH group from another molecule, and the reverse can give it back.
Next, we have the NAD+ <-> NADH reaction:
As you can see, we simply attach or remove a single hydrogen atom, as well as exchange some electrons.
2.2. Glycolysis
Glycolysis converts a glucose molecule into two molecules of pyruvate. It can be split into two stages. In the first stage, we convert two ATP molecules into ADP, and split the glucose molecule in half.
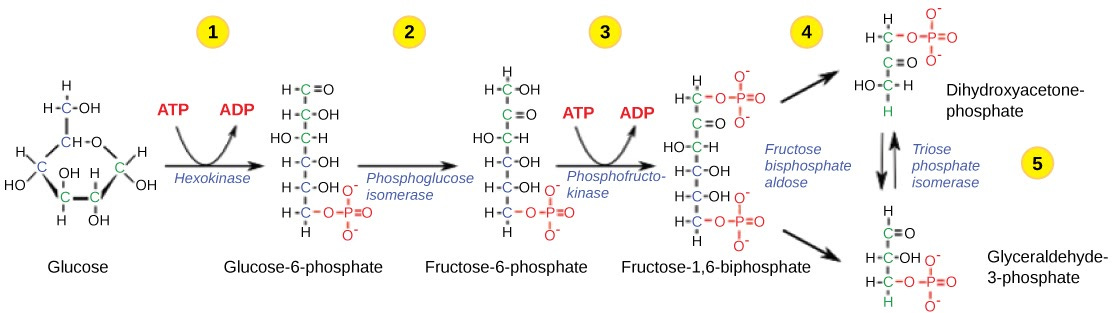
Now let’s consider what would happen if our ectoplasmic atoms vanished halfway through this stage. Phosphate groups would be chopped off to drift away alone, but they are already present in the cellular matrix: this is completely inconsequential. ADP molecules acquire an OH group from the glucose; this means that if it vanishes, that end of the ADP molecule will convert from phosphate to phosphite. This is a moderate problem, as phosphite is different chemically from phosphate: for example, bond angles are different. Wherever this would make that ADP molecule useless, or simply will modify it’s functioning (e.g. reduce efficiency), I can’t say. What I can say is that the bonds between the phosphate groups are weak, break easily, and ADP can turn into AMP (ATP = three groups, ADP = two, AMP = one); this would break off the phosphite to float in solution, and eventually to be recycled by the cellular mechanisms.
If ADP gets reduced to ATP before unsnapping, dangerous ectoplasmic OH group would likely turn into a water molecule within solution, which would then get turned into a free hydrogen atom.
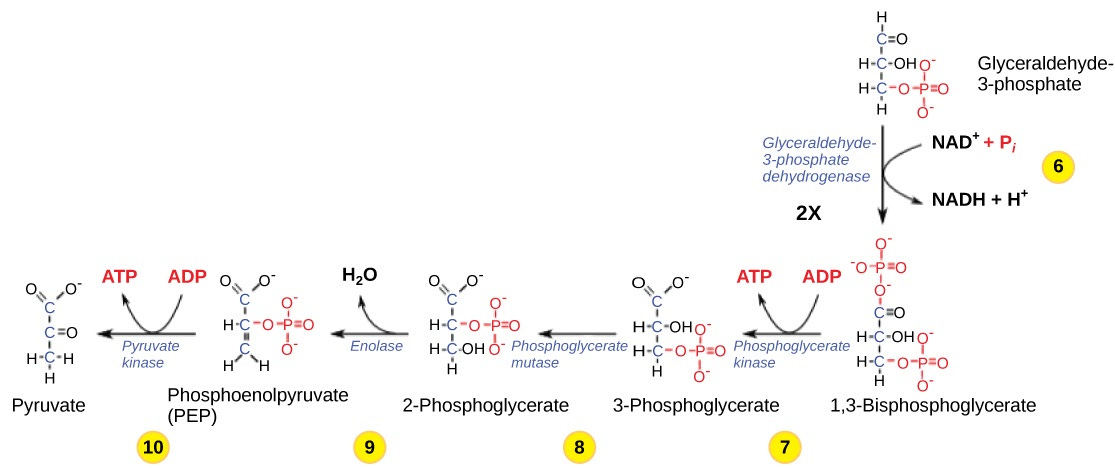
In the second part of glycolysis, first, a phosphate group from the solution gets added to the molecule, and NAD+ is reduced into NADH and a free hydrogen ion. If our molecule vanishes at this point, we are fine: the free hydrogen ion would vanish from the solution, and NADH would get converted into NAD, which would swiftly acquire the positive charge from the solution. Phosphate group would drop into solution, which already contains many of them: we would thus go back to the status quo with no damage.
Then, two ATP molecules are recovered, producing one water. Because recovery of ATP involves us getting back the phosphate group we previously attached, new ATP molecules will not have any ectoplasmic atoms in them. The water molecule should be fully ectoplasmic, and so would vanish with no damage.
Overall, glycolysis is pretty safe as far as reacting with ectoplasmic matter goes. So far so good, right?
2.3. The Krebs cycle
Krebs cycle converts newly created Pyruvate molecules into more ATP and NADH.
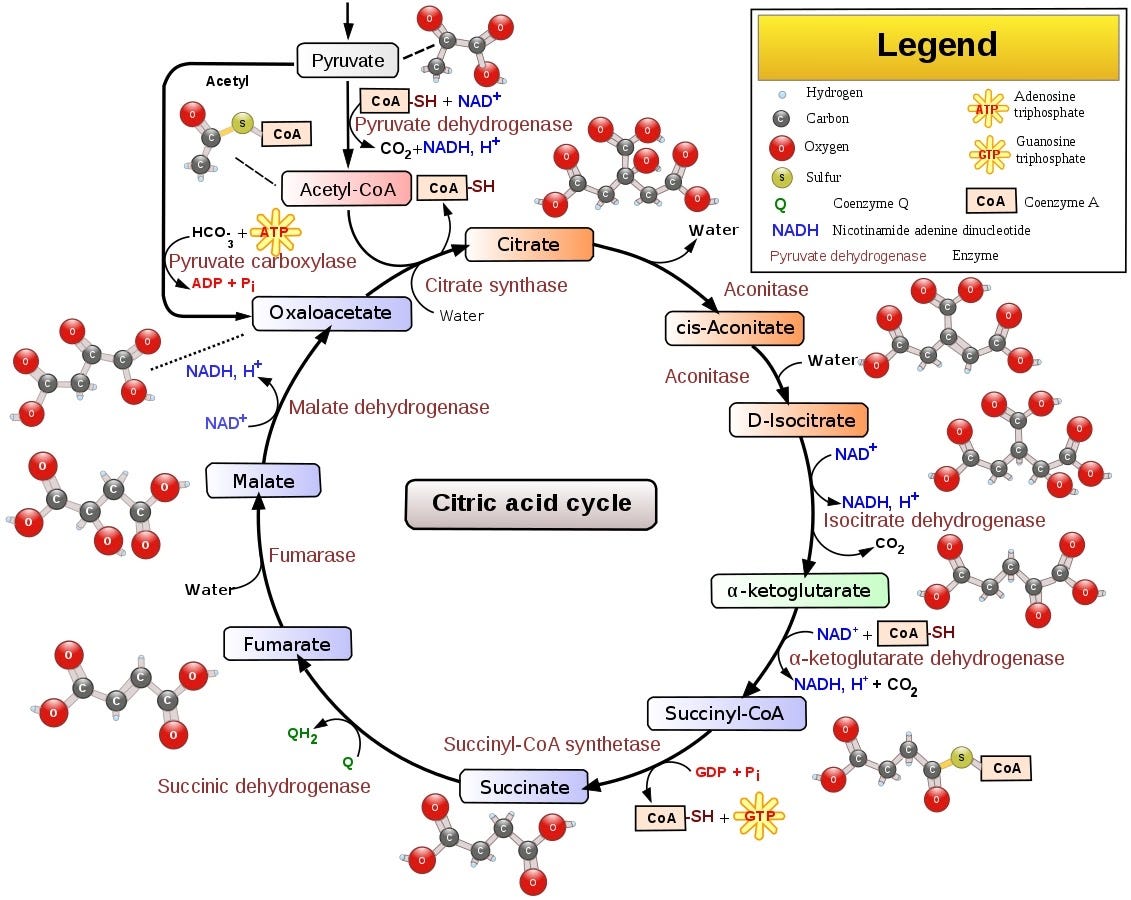
This is where we run into issues. As you can see, pyruvate comes into the cycle from the top as a result of glycolysis, and so is fully ectoplasmic. We can neglect the conversion directly from pyruvate to oxaloacetate for now, and trace the ectoplasmic atoms through the cycle. If we do that, we find that one ectoplasmic atom is left in oxaloacetate after one full run through the cycle. In the followup cycles, there is a chance it gets removed; but if we keep pumping more ectoplasm into the cycle from ectoplasmic glucose, the overall proportion of molecules with ectoplasmic atoms will grow. This means that once ectoplasmic pyruvate enters the Krebs cycle, molecules it has interacted with become “poisoned” - they will contain some ectoplasmic atoms until enough cycles pass with no ectoplasmic input for them to be leached out. If unsnapping happens before it is gone, molecules containing it would be ruined, and would thus have to be cleaned up by standard cell cleanup mechanisms.
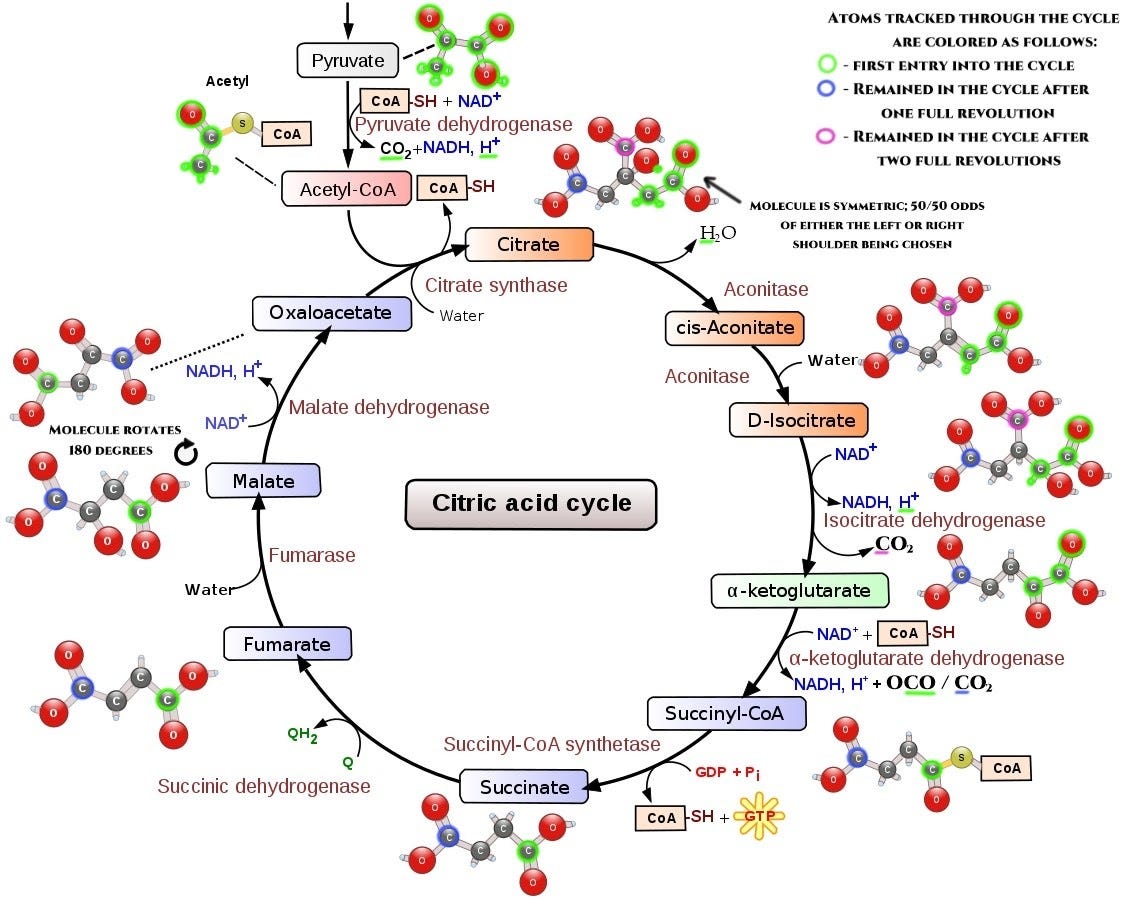
Second problem is that the Krebs cycle isn’t like the cycle of a car engine. This isn’t a single molecule being passed directly from one step to another. Instead, all of these molecules are freely floating in the cellular matrix in some concentration; every individual enzyme at every step of the cycle grabs one molecule from the common pot, modifies it, and throws the new molecule back in. How quickly they do this is determined by the concentrations of respective compounds. This means that every step in the cycle only moves carbon one step down the chain; and how likely it is to move each of the steps will depend on the concentration of the molecules with ectoplasmic carbon already in solution. And this means that carbon will spend a long time poisoning the cycle.
This wouldn’t be so bad if these molecules were just floating in some enclosed space where we could count them and determine how quickly our ectoplasmic atoms go through the cycle. But they get used all over the place, in different biochemical interactions; this means any given molecule with an ectoplasmic atom is just as likely to go to the next step of the cycle as to be used elsewhere, in a completely different cellular mechanism, in an expanding web of interactions I can’t possibly trace.
This means that after our ectoplasmic food molecules enter the Krebs cycle, there is very little I can say about what happens. Cycle poisoning effect will be real. Most of the ectoplasmic matter that actually goes through the cycle would be converted into carbon dioxide and water, and the cycle will have a self-cleaning effect that would expel ectoplasmic atoms from it as it’s being run with normal glucose as input. Some of these poisoned molecules would go on to be used in other parts of the cell metabolism, ending up who knows where and doing damage when unsnapping happens; but I can’t say whether that’s a large percentage of them or a small one, and how serious the damage would be when unsnapping happens.
3. Transmutation sickness
Having discussed chemistry, we can move on to discussing the consequences for biology. The main one is transmutation sickness, an analogue of transfiguration sickness from hpmor. It is an inevitable consequence of applying the reasoning we derived in section 1 to the insides of living creatures. I call it Transmutation Sickness instead of Summoning Sickness for two reasons: first of all, name similarity with hpmor, and second, we will see that a very similar effect happens when we consider transmutation spells in a later post.
Poster child of transmutation sickness is what happens when you eat conjured food, for example from a Mount spell (horses being edible and all). Imagine the entire process of digestion: acids and enzymes in the stomach would break the food down, letting it be slowly absorbed into the walls of your intestines. Blood would then move absorbed nutrients to the various cells in your body, where the nutrients would go through the Krebs cycle, or will be incorporated into the cellular structure. Then, after the spell expires, all of those atoms would instantly disappear.
To understand the effects of this, we would first have to consider where the food atoms are going in your body. I see three primary destinations:
Storage - some of the matter from food is simply stored, for example in fat cells, for future usage. I would also classify water in your veins into this class, for reasons that will become clearer later. If food hasn’t been absorbed by your intestines yet and is mostly just sitting around, it goes in this category too.
Cell construction and structure - cells in your body go through a continual process of renewal, and so some of the nutrients in the food will end up being used as part of the fundamental structure for cells.
Energy production cycle - most of the food is used to produce energy within cells by means of the Krebs cycle, and that’s where most of the food atoms should go.
3.1. Food absorption
Before ectoplasmic molecules can wreck all sorts of havoc on your system, they have to be absorbed into your blood. This takes some time. It’s hard for me to find figures on how quickly nutrients reach your cells, but a good proxy for that should be the time when your blood sugar rises after a meal. Typically, that figure is within 15-30 minutes, though it can be as little as 5 if you are eating pure sugar.
After the food begins being absorbed, it would continue slowly releasing nutrients into your blood over the next hours. Full absorption can take as long as several days, depending on the exact food.
This means that if you eat something ectoplasmic that doesn’t last longer than 15 minutes, it’s effect on your system will be effectively zero. And vice-versa, if food lasts long enough to be absorbed, it will continue actively poisoning your system for as long as several days.
3.2. Food storage
Making food molecules vanish from storage should be mostly safe; for water, it would mostly materialize as instant dehydration, proportional to the fraction of the water in your body that vanished. In humans, ~50-70% of body weight (40 - 50 kilos assuming 80 kg body weight) is water, and you lose a couple liters of water per day (probably around 5% of your water weight). It stands to reason that your water intake should roughly be equal to water loss, so on most days you would drink about a couple liters (split between drinks, water from food, and water produced in the Krebs cycle). If you were to drink exclusively summoned water during the day, you would expect % of summoned water in your body to reach 5% after one day, 10% after a second day, and so on.
After that water disappears, you will be dehydrated. According to wikipedia, a loss of 5% of your water weight is tolerable, 7% causes dizziness, above 10% causes “severe thirst and physical and mental deterioration”, and a loss of between 15% and 25% causes death. We can thus surmise that drinking exclusively summoned water for one way should be fine, doing it for two would cause strong backlash, and going above 4 is acutely life threatening if summoned water vanishes before you can replace it with normal water.
For fat, it may deplete your fat resources if you had stored any ectoplasmic food there, but it should not be critical: my impression is that your body does not really tap into reserves all that much, so the storage of food molecules should be minimal in the first place. To store a significant amount of ectoplasm in your fat cells you would need to do multiple cycles of fasting while eating exclusively summoned food: this is not realistic.
One major scenario where this changes is if not the entire molecule in storage is ectoplasmic, which is particularly important for water. That would cause oxidative stress, as discussed in section 1.
3.3. Cell construction
Analyzing what happens to food molecules that get used in cell construction is difficult, given the great variety of structures in the human body. Different tissues get replaced at different rates, need different materials to do so, and that replacement changes differently in response to caloric bottlenecks.
Nonetheless, we can say something. We have data on how quickly different tissues in your body get replaced; using this data, we can make a (perhaps reasonable) assumption that the amount of summoned molecules used in any particular tissue is going to be proportional to the percentage of the time you have been eating summoned food, relative to the half life of that tissue. Looking at the data, we can see that the shortest half lives come from the cells in the intestinal tract, on the order of a week or two. Based on that, a day of eating exclusively summoned food should lead to perhaps 5-10% of your intestines being affected by the ectoplasmic replacement, and thus losing function after unsnapping. Symptoms of that should be similar to radiation poisoning, as the intestinal tissue is one of the first organs to be affected. Longer periods of eating summoned matter would lead to progressively worse symptoms, until enough of your intestinal tract collapses at once that death is inevitable.
Overall, symptoms of this kind of transmutation sickness should be digestive problems, blood in your stool, trouble breathing, and a temporary drop in your immune system and clotting factors. I think that problems related to the digestive tract and the lungs should kill you faster than anything else, so replacement rate of other tissues will not have a chance to become relevant.
3.4. Krebs cycle
I have already described Krebs cycle from a chemical perspective. Now it’s time to put it into the context of biology and food ingestion.
The key question here is as follows. We know that the molecules engaged in the Krebs cycle are fairly easy to disrupt via “poisoning” with ectoplasmic atoms. However, we don’t have a sense of scale yet. Is this a long-term problem, or will it show up immediately after we ingest even a single summoned sandwich?
As I’ve said in section 2, it’s very hard to reason here, because Krebs cycle hooks into a lot of other processes in the body. But we can try to make a Fermi estimate. We know that every molecule of glucose makes the Krebs cycle run twice. By comparing the total weight of all the compounds engaged in it to the total weight of food ingested, we can get a handle on the scope of the problem.
We have nine molecules here: Citrate, cis-Aconitate, D-Isocitrate, alpha-ketoglutarate, Succinyl-CoA, Succinate, Fumarate, Malate and Oxaloacetate. This great piece of research (See figure 2, part C) provides us with the cellular concentration numbers for all of these compounds except Oxaloacetate and Succinyl-CoA.
Concentration of oxaloacetate is not provided because, I assume, they had trouble measuring it: according to this article it is “more dilute than any other tricarboxylic acid cycle (Note: other name for the Krebs cycle) intermediate”. It is also chemically unstable.
I am not sure why succinyl-CoA is not in the data: it is relatively stable and present in measurable concentrations. For example, this paper gives a value of 0.3 - 1.4 mM. Due to the very heavy CoA tail, it is singularly responsible for most of the mass engaged in the Krebs cycle.
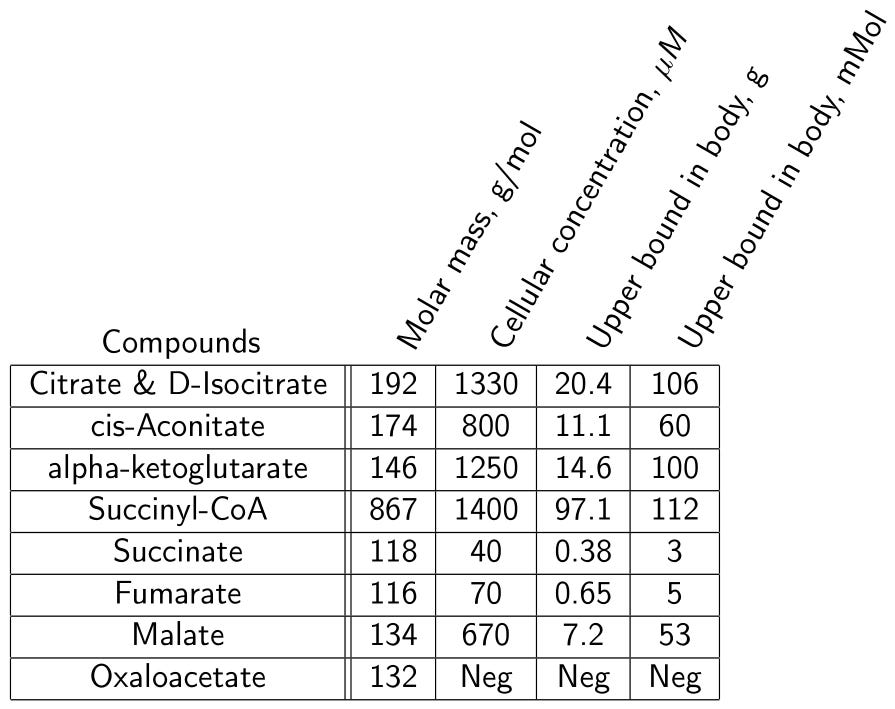
Total amount of Krebs cycle compounds in your body is, at most, 0.44 mol. One ectoplasmic molecule of glucose introduces 2 ectoplasmic carbons into the cycle, and glucose has a molar mass of 180 grams; this means that just 90 grams (weight of a small hamburger) of it would be enough to poison all the compounds currently engaged in the Krebs cycle twice over. Of course, these are just averages: factors like local availability of compounds, import and export of them to or from other metabolic cycles, and degree of homogeneity in the mitochondrial matrix will also affect things. But overall, I think we can conclude that after even a fairly minimal amount of ectoplasmic food starts being passed through the Krebs cycle, the vast majority of the mitochondria in your body would get completely poisoned by the ectoplasmic carbon. When unsnapping happens, all of the poisoned molecules would break in half and become unusable for their purpose in the cycles; energy production in your cells would thus suffer an immediate and severe disruption.
On a positive note, it should be similarly easy to clean up the cycles by sustained ingestion of normal glucose over the course of a day.
How lethal would this be?
It is impossible to find any data on what such a widespread disruption would do to you, because it is literally impossible in real-life physics. Nonetheless, we can try to get a handle on the consequences by looking at other kinds of disruptions of things involved in the Krebs cycle, and try to guess how bad it can get.
Pyruvate carboxylase is an essential enzyme: it is used in the production of glucose and in conversion of Pyruvate into Oxaloacetate. Genetic disorders leading to a deficiency in this enzyme are almost invariably fatal in early childhood.
Sodium fluoroacetate is a toxic chemical that, when ingested, binds tightly to Aconitase, preventing conversion of citrate into aconitate and thus halting the citric acid cycle. It is lethal to humans, with LD50 measured in milligrams per kilo. Symptoms begin approx 30 minutes after ingestion, which, unless I am mistaken, happens to be exactly when it should be reaching your cells.
Finally, the main way asphyxiation kills you is by preventing hydrogen recovery in the electron transfer chain, and thus preventing ATP molecules from being regenerated.
Long story short, anything that prevents the Krebs cycle from functioning is extremely lethal extremely quickly.
Could we recover from an unsnapping disruption before we die?
Let’s say that just half of all the molecules involved in the cycle have been destroyed. We can also assume that the body has maybe two minutes before it runs out of previously generated ATP - a typical time needed to suffocate. ATP is generated at the rate of 9e20 molecules per second; this comes out to 2 mMol of glucose being imported into your cells per minute. Molecules in the Krebs cycle have about the same molar weight as glucose; this means that If half of them have been destroyed and will need to be rebuilt from the ground up, we will need to import on the order of 200 mMol of glucose into your cells just as building materials. Increasing the import of glucose by two factors of magnitude seems highly unrealistic to me. I believe this means that you will not be able to regenerate the substrate necessary for the Krebs cycle by the time you run out of ATP, and thus will die.
To summarize, if you have eaten a moderate amount of ectoplasmic matter as food, and it got absorbed into your cells as glucose before unsnapping, you will likely die in a manner similar to asphyxiation or action of nerve agents. “Purging” with normal food should be reasonably effective at minimizing the effects of ectoplasmic poisoning, assuming you can manage to fit in an entire digestive cycle before the spell ends.
3.5. Gas exchange
Gas exchange is the process of breathing on a chemical level: oxygen being absorbed by the red blood cells, delivered to other cells, and carbon dioxide being delivered from the cells back to the lungs. It is the second major biochemical pathway for ectoplasmic molecules inside of your body.
Key protein involved in gas exchange is hemoglobin. It sits inside of the red blood cells, and can bind to three key molecules - oxygen, carbon dioxide, and carbon monoxide. You can see how they bond to a hemoglobin plaque below. This binding is three-dimensional: hemoglobin is a “plate” with the bound molecule sticking out like a spike in the middle of it.
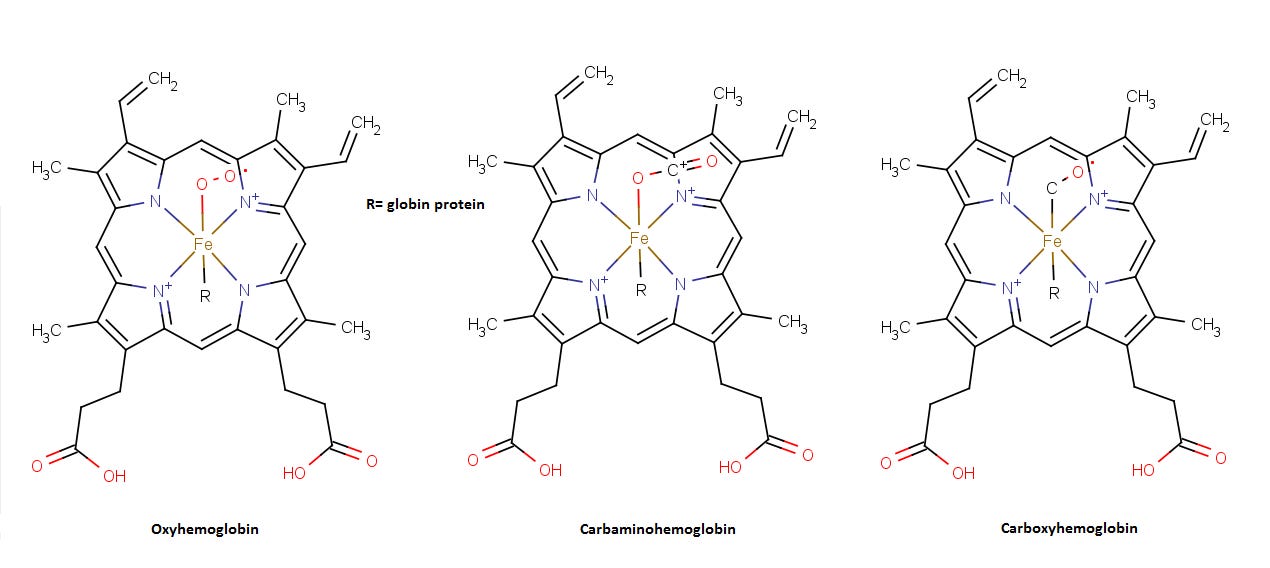
Carbon monoxide bond is the strongest of the three, which is why carbon monoxide poisoning is so deadly - after it binds to hemoglobin it takes a while (half life ~5 hours when breathing air, ~1 hour on pure oxygen) for it to break off, and in the meantime hemoglobin can’t transport oxygen.
3.5.1. Fully ectoplasmic oxygen
Let’s now consider what happens if the molecule bound to hemoglobin is ectoplasmic. If the entire molecule is ectoplasmic, then it vanishes harmlessly, and hemoglobin is ready to take in more oxygen again. In the case of carbon dioxide or monoxide, this is entirely harmless. In the case of oxygen, this would mean the red blood cell can’t deliver any oxygen to the cells; I believe this should be largely harmless too, even in a limiting case where you are breathing 100% ectoplasmic oxygen. My logic goes like this: it takes about a minute for your blood to circle the body; humans are fine holding their breath for a minute, meaning your body is fine with not getting any new oxygen for a minute. Let’s say that you have been breathing exclusively ectoplasmic oxygen for a while so it is bound to all your red cells; after it unsnaps, all of your blood would be deoxygenated, but if you can immediately get access to regular air, you should be able to re-oxygenate your blood before you start to run into major issues.
One problem here would be that as you come off the spell you would have no idea that your blood is out of oxygen. Your body uses carbon dioxide concentration to figure out if it should be breathing harder, which won’t spike just because your blood happens to have zero oxygen all of a sudden. This means at a time when you should be breathing very rapidly (as rapidly as if you have just spent a minute or more holding your breath) you will feel completely fine, up until the point where you collapse. Without oxygen, your brain will no longer be able to recover NADH into NAD+ and thus won’t be able to run the Krebs cycle to produce energy necessary for your nervous system to stay alive. As long as you keep breathing normal air though, I think you should come back to consciousness. One way to avoid this would be to start breathing normal air before the spell ends, or to start deliberately hyperventilating as it’s ending.
3.5.2. Semi ectoplasmic molecules
If the molecule is only partly ectoplasmic, the effects of unsnapping are somewhat different. I’ve outlined the relevant reactions in section 1.4, formulas 7, as well as their recombinations in section 1.5. The four potential resulting compounds are molecular oxygen, atomic oxygen, atomic carbon, and carbon monoxide.
Oxygen. Molecular oxygen would bind to hemoglobin safely. Atomic oxygen, on the other hand, would lead to damage; due to its high reactivity, I think it’s sensible to assume that that bonding spot on the hemoglobin molecule will stop being functional due to damage.
Carbon. I can’t find any data regarding how well pure carbon bonds to hemoglobin; overall, I think it should bond worse than carbon monoxide. Bonding of carbon monoxide is influenced by that molecule being polar, which is not the case for a single atom of carbon. If that is the case, carbon should fall off safely. If it isn’t, it’d likely get oxidized to CO2 next time it passes by the lungs.
Carbon monoxide will likely immediately bond with hemoglobin. See section 3.5 regarding the half life necessary for it to unbind.
Overall, the only truly dangerous case here is a reaction with molecular oxygen, which will damage the hemoglobin molecules. To get a handle on how serious of a problem this may be, let’s say that half of your blood is carrying carbon dioxide, and the other half is carrying oxygen. Let’s further assume the worst case scenario: we are running on 100% ectoplasmic glucose. Looking at the Krebs cycle, this means 2/3 of the carbon dioxide will be partly ectoplasmic and will release atomic oxygens, and 1/3 will be fully ectoplasmic. In that case, about one third of the hemoglobin in our blood would be damaged (2/3 times 1/2).
If we look at the medical tests for hemoglobin, normal results for men are around 150 grams per liter. Losing one third of that would put us down to 100 grams of functional hemoglobin per liter; well into what is considered to be moderate anemia. It would take a while to pass, as a full half of all of the red blood cells in your body would need to be replaced. You are probably looking at a period of half a year to a year over which your symptoms would gradually get better.
Remember that this is the worst case scenario: the more real glucose is going through your metabolism at the time of unsnapping, the lesser the damage will be, proportionally.
3.5.3. Endpoint delivery
Hemoglobin isn’t just hanging on to gas molecules for no reason; it is transporting them somewhere. For carbon dioxide and carbon monoxide this is the lungs, where they are breathed out safely. For oxygen, it is moved to the cells, where it interacts with four NADH in the electron transfer chain to produce four NAD+ and two molecules of water. Breathing in ectoplasmic oxygen thus would thus lead to production of H2O, and thus atomic or molecular hydrogen when unsnapping happens; refer to section 1.5 for analysis of the consequences of hydrogen production.
Overall, transport of fully ectoplasmic oxygen should be fairly safe, with a side effect of oxidative stress due to atomic hydrogen production. Transport of carbon dioxide from the Krebs cycle carries some risks of anemia, but is overall safe, or at least much safer than the problems caused by the Krebs cycle itself.
3.6. Hydrogen pressure
There is a more dangerous point I haven’t brought up in the previous section when it comes to the production of hydrogen. To explore it, let’s begin by figuring out how much hydrogen will be produced.
An average person exhales about a kilo of CO2 a day, though with exercise it can be up to 8 times more. Molar mass of CO2 is 44 g/mol, so this comes out to 22.7 moles per day. We know that the Krebs cycle produces three molecules of carbon dioxide per run; we also know it produces twelve hydrogens (two from glycolysis, plus eight in pairs of NADH + H+, and two for enzyme Q). Twelve moles of atomic hydrogen will recombine with oxygen in the electron transfer chain to produce six moles of water; assuming we are breathing fully ectoplasmic oxygen, this will produce up to six moles of H2. Let’s conservatively say that three moles would recombine with nearby organic molecules, leading to oxidative stress. If that assumption is roughly correct, then for every mole of carbon dioxide produced, we produce a mole of molecular hydrogen, for a total value of approximately 22.7 moles in your entire body. This hydrogen will be pretty widely spread out throughout the organism, because it has been produced within the mitochondrion of every individual cell.
Some of it would be safely dissolved in the cellular matrix. Maximum solubility of hydrogen is 0.0016 grams per liter of water; this comes down to about 0.0008 moles per liter. Assuming we are working with a 70 kilo human, their body could dissolve no more than ~ 0.056 moles of hydrogen total. The rest of the hydrogen will immediately start forming bubbles.
22 moles worth of bubbles to be precise. How much is that?
Conveniently enough, most simple gasses are quite similar when it comes to their properties under pressure. For example, one mole of most gasses takes up about the same volume of ~25 liters, at atmospheric temperature and pressure. Times 22, this means our hydrogen wants to occupy about 567 liters, compared to the measly 70 liters of your body.
Your body can withstand some internal pressure - for example, one atmosphere of difference should be survivable. But stuffing 567 liters of gas into your body will produce much more. The only possible result here is an immediate explosion, splattering your body all over the nearby walls.
Fine, so one full day of breathing ectoplasmic oxygen is way too much. What’s the maximum amount of time we could breathe it without dying instantly once the spell ends?
Well, if we are down to one hour of breathing, then the generated volume would be 24 times smaller - only about 23 liters. If we assume it can be compressed to double atmospheric pressure, this would be only 11.5 liters, making all of the cells in your body expand by about 10-20%. This still kills you.
If we reduce the time down to 5 minutes, then your cells would only have to expand by 1.5% to accommodate that pressure. That seems like a potentially survivable value to me, though I don’t have data to back up this assertion. Of course, there are other problems besides the cellular pressure. Some of that water would have no doubt diffused into your blood - from the red blood cells, cells neighboring the blood vessels and so on. If we assume that this semi-ectoplasmic water was roughly uniformly distributed throughout your entire body, then your veins would suddenly need to cope with about 100 millilitres of hydrogen gas. Given that only about 2 milliliters of gas are enough to cause a heart attack, I am not rating your survival chances highly.
This value may be much smaller if the water does not diffuse into your veins quickly enough, leading to an abnormally small concentration of semi ectoplasmic water in the blood. In that case, hydrogen would probably start slowly leaching out of your cells into the blood, and may get safely removed through your lungs over a prolonged period of time. This would still almost certainly cause symptoms, very similar to the ones caused by decompression sickness because the causes of the symptoms will be very similar (only with hydrogen instead of nitrogen). Based on solubility numbers for nitrogen, real-life bends involve tens of millilitres of gas per liter of water - about the same as the amount of hydrogen generated from the semi-ectoplasmic water in our case.
Treatment for such “ectoplasmic decompression sickness” would be very similar to the treatment for regular decompression sickness: keep the person in a high pressure environment until the gas has enough time to dissolve and diffuse out of their body. Breathing ectoplasmic oxygen when hundreds of meters underwater is much safer than on the surface.
Of course, all of these calculations were made under the assumption of normal sedentary energy consumption. If you are actively moving around (such as during combat), then the amount of CO2 generated - and thus amount of oxygen inhaled, and water produced - is up to 8 times higher.
This all means one thing: there is basically no safe amount of ectoplasmic oxygen that you can realistically inhale without dying. This, in turn, means that spells like Air Bubble must create permanent matter, or they would risk killing their users.
This concludes the discussion of ectoplasmic chemistry. Next post in the series would deal with summoning blueprints, and how they limit the summoning spells available in practice.
Meanwhile, if you enjoy what I write, you can subscribe to receive updates by email:
Licensing
Because this article uses images licensed under creative commons, it is licensed under CC BY-NC-SA 4.0 to the general public.